A systematic literature review of clinical trials and therapeutic applications of ibogaine
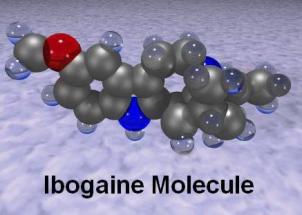
Addiction Psychopharmacology
Addiction Research Articles
Ibogaine Research
Ibogaine Research and Articles
Psychedelic & Interventional Medicine
Ibogaine
Overview
Originally Published: 07/22/2022
Post Date: 04/07/2023
by Patrick Köck, Katharina Froelich, Marc Walter, Undine Lang, Kenneth M. Dürsteler
Attachment Files
PDF. A systematic literature review of clinical trials and therapeutic applications of ibogaine
Summary/Abstract
A systematic review focusing on clinical data and therapeutic interventions involving ibogaine and noribogaine.
Content
Highlights
- This review provides an overview of the therapeutic uses of ibogaine and noribogaine
- Data suggests effectiveness in the treatment of substance use disorders
- Due to potentially lethal consequences, rigorous medical settings may increase safety
- Global consensus regarding legal status of ibogaine and noribogaine is absent
- Eligible individuals may benefit from rapid-onset therapies like ibogaine
Abstract
Background
Iboga and its primary alkaloids, ibogaine and noribogaine, have been of interest to researchers and practitioners, mainly due to their putative efficacy in treating substance use disorders (SUDs). For many SUDs, still no effective pharmacotherapies exist. Distinct psychoactive and somatic effects of the iboga alkaloids set them apart from classic hallucinogens like LSD, mescaline, and psilocybin.
Aims
The study team performed this systematic review focusing on clinical data and therapeutic interventions involving ibogaine and noribogaine.
Methods
The team conducted a search for all publications up to December 7, 2020, using PubMed and Embase following PRISMA guidelines.
Results
In total, we identified 743 records. In this review, we consider 24 studies, which included 705 individuals receiving ibogaine or noribogaine. This review includes two randomized, double-blind, controlled clinical trials, one double-blind controlled clinical trial, 17 open-label studies or case series (including observational or retrospective studies), three case reports, and one retrospective survey. The published data suggest that ibogaine is an effective therapeutic intervention within the context of SUDs, reducing withdrawal symptoms and craving. Data also point toward a beneficial impact on depressive and trauma-related psychological symptoms. However, studies have reported severe medical complications and deaths, which seem to be associated with neuro- and cardiotoxic effects of ibogaine. Two of these fatalities were described in the 24 studies included in this review.
Conclusion
Treatment of SUDs and persisting comorbidities requires innovative treatment approaches. Rapid-onset therapies such as the application of ibogaine may offer novel treatment opportunities for specific individuals. Rigorous study designs within medical settings are necessary to warrant safe application, monitoring, and, possibly, medical intervention.
Keywords
1. Introduction
Iboga and its main active alkaloids, ibogaine, and noribogaine, as well as structurally related alkaloids, have gained increasing scientific attention over the last decades, mainly due to their proposed “anti-addictive” properties. Although we do not yet fully understand their complete pharmacological mechanisms, available data suggest efficacy in the treatment of opioid use disorder (OUD), cocaine use disorder (CUD), and other substance use disorders (SUD). Previous research points out the necessity of conducting safe study designs and controlled clinical studies (dos Santos et al., 2016). Treatments with ibogaine are considered safe when properly medically supervised. However, several case reports have been published about fatalities or adverse events associated with the ingestion of iboga plant material or ibogaine. Global medical and legislative regulatory consensus is absent. Although classical hallucinogens have been studied and are currently under investigation for a wide array of psychiatric conditions, including SUDs (Bogenschutz & Johnson, 2016; Bogenschutz & Ross, 2018), controlled clinical trials with ibogaine are still scarce.
The main aim of this systematic review was to identify all human studies on the use of ibogaine or noribogaine with explorative or therapeutic intention that took place up to December 7, 2020, and assess effectiveness outcomes of included studies.
1.1. Ibogaine
Ibogaine is an indole alkaloid found in the shrub Tabernanthe iboga (T. iboga), commonly known as iboga (Alper, 2001) and in the plant Voacanga africana (Kikura-Hanajiri et al., 2009). Vatharanthus, Corynanthe, and Aspidosperome genera also produce iboga alkaloids. Those plants belong to the family of the Apocynaceae (Kinghorn, 2017). These plants contain several alkaloids that are being researched or have yet to be identified. According to recent publications, about 100 natural or synthetic iboga indole alkaloids are defined based upon their typical ibogamine skeleton (Lavaud & Massiot, 2017). Researchers have investigated some of these compounds for different medical purposes and pharmacologic profiles (Cameron et al., 2020; da Silva Brum et al., 2016; Freissmuth et al., 2018; Gómez-Calderón et al., 2017; Ishikawa et al., 2008). From a psychiatric perspective, the use of T. iboga and its major alkaloid ibogaine seems compelling due to its putative anti-addictive properties (dos Santos et al., 2016; Winkelman, 2014). Researchers have explored other therapeutic indications, such as the treatment of psychological trauma and depressive symptoms, in recent years. In West Central Africa, “iboga” or “eboka” has traditionally been used by the indigenous Bwiti religion to conduct ceremonial rites. Eboka designates the root bark shavings of the Tabernanthe iboga plant, which is the major form used in rituals in Africa (Antonio et al., 2013; Fernandez & Fernandez, 2001). In the literature, the use of iboga plant material is referenced commonly by its ethnobotanical abbreviation T. iboga, whereas ibogaine refers to the indole alkaloid form (Alper, 2001). Initial studies looked at ibogaine's effects on the central nervous system and its cardiovascular actions. Ibogaine was marketed in France as Lambarene® and licensed as a “neurostimulant”. France withdrew Lambarene® from the market in 1966 when the sale of ibogaine-containing products became illegal in that country (Glue, Winter, et al., 2015). The United States assigned ibogaine Schedule I classification, and the International Olympic Committee banned it as a potential doping drug (Mačiulaitis et al., 2008). Ibogaine is used in medical and nonmedical settings to treat addictive disorders, most commonly for opiate detoxification (Alper et al., 2008). In 2012, the U.S. National Institute on Drug Abuse (NIDA) supported the production of 18-methoxycoronaridine (18-MC) and pre-clinical studies investigating pharmacotherapeutic applications for SUDs. 18-MC is a synthetic iboga alkaloid (National Institutes of Health, 2012), which seems safer than ibogaine regarding cardiotoxicity (Corkery, 2018). Furthermore, research has found tabernanthalog, a recently engineered, non-hallucinogenic, non-toxic ibogaine analog, to produce antidepressant-like effects and reduce alcohol- and heroin-seeking in rodents (Cameron et al., 2020).
1.2. Theories about pharmacological mechanisms
Animal models in addiction research have shown that ibogaine, noribogaine,18-MC, and the novel analog tabernanthalog decreased self-administration or drug-seeking of various addictive substances (Cameron et al., 2020; Glick et al., 2000; Mačiulaitis et al., 2008; Pace et al., 2004; Rezvani et al., 2016). Multiple receptor systems are involved in this decrease, but we do not yet fully understand the exact mechanisms. Nevertheless, researchers have established some theories to explain the observed effects. Results from cell culture and animal models suggest that glia cell line-derived neurotrophic factor (GDNF) up-regulation contributes to reduced ethanol self-administration (Carnicella et al., 2010). N-methyl-d-aspartate (NMDA) receptor antagonism may also be involved in the effects of ibogaine (Baumann et al., 2001). Radioligand binding assay experiments suggest that ibogaine blocks nicotinic acetylcholine receptors (Arias et al., 2010). Research has proposed the blockage of nicotinic α3β4 receptors in the medial habenula as the potentially cause for the observed effects of reduced alcohol and nicotine intake after oral 18-MC ingestion (Glick et al., 2002). Other theories suggest modification of opiate receptor-mediated signaling as a possible mechanism. While serotonin (5-HT) receptor agonism and serotonin transporter (SERT) inhibition might be involved in hallucinogenic or putative antidepressant effects, its exact role remains unclear (Glick et al., 2001). Research has recently described the pharmocochaperone-activity of ibogaine and noribogaine on the SERT and the dopamine transporter (DAT) (Freissmuth et al., 2018). While ibogaine and noribogaine interact with several central nervous receptors, studies have reported the strongest affinities of ibogaine for the sigma2-receptor, the opioid receptors, SERT, and DAT (Preedy, 2016; Ray, 2010). However, Antonio et al. (2013) demonstrated that opioid agonism does not seem to account for the observed effects of the iboga alkaloids in opioid withdrawal. κ-opioid receptors (KOPR) may also play a specific role. Dynorphin and the KOPR system are essential in CUD (Bidlack, 2014). Research has proposed that pharmaceuticals displaying agonist and antagonist qualities upon KOPR can treat CUD (Maillet et al., 2015), and noribogaine seems to possess these qualities. High SERT affinity, especially documented for noribogaine, might explain sustained antidepressant effects. Considering affinity data (Ray, 2010), the specific multi-receptor targeting and the long action of ibogaine/noribogaine might be keys for disrupting neuronal circuits involved in SUDs. The involvement of multiple receptor systems and pharmacokinetic mechanisms might explain postulated effects upon substance craving (via DAT inhibition, NMDA antagonism), withdrawal symptoms (via opioid receptors), and post-withdrawal depression (via prolonged SERT inhibition). GDNF expression may play a role in neuroplasticity and hence foster behavioral change. Hallucinogenic properties (possibly via 5-HT2A agonism, sigma receptor activity) might be necessary for insight and meaningful experiences. Pharmacochaperone activity might also be involved in long-term behavioral changes.
1.3. Pharmacokinetics and pharmacodynamics
The majority of ibogaine studies on humans have looked at the drug's effects on SUD symptoms. The administration route of ibogaine in these studies was oral (p.o.). Single ibogaine doses in earlier studies ranged from 500 to 800 mg (dos Santos et al., 2016). These doses yielded plasma concentrations Cmax for ibogaine and noribogaine between 30 and 1250 ng/ml and 700–1200 ng/ml at Tmax around 2 h and 5 h after ingestion, respectively (dos Santos et al., 2016; Glue, Lockhart, et al., 2015; Glue, Winter, et al., 2015). Blood concentrations of ibogaine and noribogaine show high interindividual variability. Poor metabolizers showed higher blood concentrations of ibogaine relative to noribogaine over time. Extensive metabolizers have shown an inverted blood concentration profile within measurement times, with relatively higher blood concentrations of noribogaine due to faster metabolization of ibogaine (Mačiulaitis et al., 2008). One research study has documented hepatic metabolism, primarily via CYP2D6, followed by CYP2C9 and CYP3A4 (Obach et al., 1998). Storage of ibogaine in adipose tissues suggests a protracted ibogaine/noribogaine release with time (Alper, 2001). Generally, ibogaine seems to be cleared quickly from the blood, while significant noribogaine concentrations could be measured 24 h after oral ingestion (Mačiulaitis et al., 2008). Lower doses of ibogaine showed no detectable plasma levels after 4 h post-ingestion. CYP2D6 inhibition significantly prolonged Tmax and plasma availability (Glue, Winter, et al., 2015). The application of noribogaine 180 mg p.o. resulted in t½ of 24–30 h (Glue et al., 2016).
1.4. Subjective effects
Research has divided the ibogaine experience into three phases (Alper, 2001). Phase I has been described as oneiric (“waking dream”) state in which the individual experience visual and other sensory perception changes and panoramic recall of earlier life events (duration 4–8 h). After phase I, the experience changed to a subtler experience. Phase II has been described as evaluative, emotionally neutral, and reflective. Phase II lasts between 8 and 20 h. Phase III has been titled as a residual phase comprising heightened awareness, mild stimulation, and, eventually, perturbed sleep patterns. Phase III can last up to 72 h after ingestion (Alper, 2001; Glick et al., 2001). Reports suggest that ibogaine can cause a more intense psychedelic experience than previous experiments with high doses of psilocybin. Participants have mentioned insights relating to the meaning of life, the evolution of the universe, life-after-death, and feeling relieved from guilt (Heink et al., 2017).
1.5. Safety and toxicity
The literature reports several fatalities and severe toxic adverse events in humans related to ibogaine treatments. Adverse symptoms range from nausea, tremors, ataxia, and psychiatric conditions (e.g., mania, psychosis) to severe clinical effects such as seizures, comas, pulmonary difficulties, and fatal outcomes (dos Santos et al., 2016; Litjens & Brunt, 2016; Schep et al., 2016). Cardiotoxicity and QT prolongation, which increases the risk for Torsade de Pointes, pose a significant problem. Ibogaine's modulatory action upon Human Related Ether-à-go-go Gene (hERG) channels seems to cause a reduction in electrical currents via potassium channels. This results in a delayed cardiac repolarization (Alper et al., 2016; Koenig et al., 2014; Koenig & Hilber, 2015). Eighteen of 33 previously analyzed fatality reports had preexisting medical conditions, such as coronary sclerosis or cardiac arrythmias. Twelve of 33 cases report concomitant drug or medication use, or the consumption of unknown origin and purity material (Corkery, 2018). Litjens and Brunt (2016) reviewed ibogaine's pharmacological profile and toxicity of ibogaine and its metabolites. Animal data showed neurodegenerative processes caused by ibogaine and to a lesser extent by noribogaine. Research has specially described excitotoxic effects on Purkinje cells in the cerebellum. Litjens & Brunt also present eight cases of cardiac abnormalities following the ingestion of ibogaine. An analysis of these cases revealed predominantly QTc-prolongation, ventricular tachycardia, and cardiac arrest as leading clinical manifestations. The study also discussed the importance of drug-drug interactions as a cause of adverse drug reactions. In conclusion of their review, the authors point to the risk of more ibogaine-related deaths and medical emergencies in the future, particularly in patients with cardiac comorbidities and concurrent medication (Litjens & Brunt, 2016). Structurally related congeners like 18-MC or tabernanthalog seem less cardiotoxic and non-hallucinogenic, but studies need to assess their clinical and therapeutic properties (Cameron et al., 2020; Corkery, 2018; Schep et al., 2016).
2. Methods
2.1. Data acquisition
The research team collected data for this systematic review according to the PRISMA guidelines for Systematic Reviews and Meta-Analyses.
2.2. Search strategy
For data acquisition, our group performed systematic electronic searches using PubMed and EMBASE. The team used the following keywords: “iboga” OR “ibogaine” OR “noribogaine” AND “withdrawal” OR “addiction” OR “dependence” OR “substance use disorder” OR “detoxification” OR “craving” OR “heroin” OR “opioid” OR “opiate” OR “cocaine” OR “alcohol” OR “cannabis” OR “tobacco” OR “nicotine” OR “therapy” OR “treatment” OR “therapy” OR “clinic”.
2.3. Eligibility criteria
Article type: The team included articles, case reports, and case-series in peer-reviewed journals. We also included book chapters, books, posters, abstracts, letters, and editorials if they were indexed in the databases mentioned above.
Study design: All kinds of study design.
Participants/sample: Human individuals.
Interventions: Administration of iboga, ibogaine, or noribogaine for investigative and therapeutic purposes.
Comparisons: Pre-post and placebo-controlled effects.
Outcomes: Substance use, SUD symptoms, depressive symptoms, and safety aspects.
2.4. Data extraction
Two independent reviewers (PK, KF) screened and assessed all electronically collected data. A third reviewer (KD) resolved discrepancies between rater PK and KF. From the articles included, our team recorded authors' names, publication date, setting (medical or nonmedical), study location (country), study type (case reports or case series, retrospective or observational studies, open-label clinical trials, placebo-controlled studies), number of participants receiving iboga/ibogaine/noribogaine, demographic data, study intention, substances used, dose, administration route, safety measures, serious adverse events, main results, and previous treatments.
3. Results
3.1. Selection of records
Following the PRISMA guidelines (Moher et al., 2009), we performed data acquisition and analysis for inclusion and exclusion of relevant literature (Fig. 1). Through the performance of database searches (PubMed, Embase), we identified 1038 records. In addition, we found four records via other sources (Research Gate). After the removal of duplicates, 743 records remained for screening. Through independent screening (PK, KF) of all records, the team excluded 710 papers (pre-clinical data, animal studies). Hence, we assessed for eligibility 33 full-text articles, and of those, we included nine. We excluded eight articles due to the absence of clinical data (Barber et al., 2020; Blessing et al., 2020; Schellekens et al., 2016) or redundant information (Alper et al., 2000; Calvey et al., 2020; Davis et al., 2018; Lotsof & Alexander, 2001; Mash, 2018). One record had not been published (Bastiaans, 2004). Finally, the team included 24 studies in this systematic review's qualitative synthesis, meeting pre-established eligibility criteria. We present the findings in Table 1 and Table 2. We split the dataset into two tables for better readability. The 24 selected studies included seven case reports (CR) or case series (CS), eight observational (OS) or retrospective studies (RS), six open-label clinical trials (OL, CT), and three double-blind, placebo-controlled clinical trials (DBPCT). All selected studies described the application of ibogaine or noribogaine. While most of the reviewed publications used ibogaine hydrochloride (HCl), some studies did not specify the exact chemical designation and we marked them accordingly in Table 2.